What’s on the menu for small Arctic animals?

The Polar cod is a key species in the Arctic. Pictured here in a buffet of its favourite food, namely zooplankton. Photo: Geir Johnsen (NTNU/UNIS).
Top image: The Polar cod is a key species in the Arctic. Pictured here in a buffet of its favourite food, namely zooplankton. Photo: Geir Johnsen (NTNU/UNIS).
Describing who eats who is important to understand how nutrition and energy move between species in marine ecosystems. Today, the field of research is flourishing like never before thanks to new technology and more advanced methods. These allow us to uncover hitherto unknown connections that occasionally shake up known paradigms in ecology.
10 August 2020
Text: Snorre Flo, PhD candidate, UNIS/UiT and the Nansen Legacy.
One of the paradigms in marine ecology is that the animals’ diet can be linked to their size. In other words, it is understood that “big eats small”, but “big” is hunted and eaten by something bigger, and so food and energy migrate up the food chain. Studies that use new methods and technology indicate that this paradigm does not necessarily provide a good understanding of reality, and that we can occasionally replace “big eats small” with “small eats big”.
How can that be?
To make sense of this, let us first start by describing a typical marine food chain in the Barents Sea. Most life so far north depends on nutrients and energy produced by single-celled algae. Every spring, when the sea ice melts and the sun returns, the algae restart their photosynthetic machineries to form energy-rich substances from CO2, water and sunlight.
Over the course of weeks, even days, the water column can go from being nutrient-poor to becoming a feast full of calories. The first course of the menu consists of single-celled algae that are rich in energy-rich sugars and fats. These substances migrate further in the food chain when the algae are eaten by single-celled animals and small zooplankton. These are then eaten by slightly larger zooplankton, which in turn are eaten by fish and birds, then seals, and finally we reach the hierarchy’s top predator, the polar bear.
This linear model has stood firm for centuries and can be used in advantage to show how the food chain is roughly connected. But we know today that nature is incredibly much more complex than the model suggests. Therefore, we are moving further and further away from the linear model, and closer to a food network where predator-prey interactions can also occur across trophic levels and size ratios. Central to this development is the use of new technology in research that allows us to uncover hitherto unknown species interactions.
Fact box: Trophic levels are used to describe the steps in the transfer of nutrients and energy in a food chain. Typically, a food chain consists of four to five steps, with the producers (in this case algae) at the bottom, and predators at the top. |
Traditional studies are limited by our level of knowledge
To understand the advantages of today’s technology, we must first look at the older, traditional methods. Previous studies of diet have been based on visually recognizing species in the stomach, in faeces or by directly observing that one species eats another. For many animals, such methods can give good indications. For others, especially when moving down to the millimetre-sized copepods, it quickly becomes a difficult task. These creatures can be so small that their behaviour is difficult to observe. And the smaller they are, the smaller the stomach. Imagine dissecting the animal and identifying all kinds of species in a stomach the size of a pinhead.
After staring into the microscope for two hours, your eyes become tired, and what you think is a species may just as well be remnants from the antenna of a krill.

Another problem with the older methods is that they require an enormous amount of knowledge. It often takes several years of practice to be able to determine the species in a water sample with certainty. It is even more difficult to recognize species from material that is more or less digested. Particularly soft animals such as jellyfish and eggs have presented problems because they end up looking like trait-less, transparent lumps of matter, only after a short duration in the stomach. That they break down quickly can also lead to underestimating the amounts of them relative to animals that are more resistant to digestion because of their inner or outer skeletons.
Molecular methods contribute to problem solving
New methods are based on studying molecules that survive longer in the stomach than a visually characteristic shape or structure. A good example can be found in a study conducted on the diet of albatrosses in South America. We have long believed that these birds feed on fish and shellfish, but by studying the DNA in the albatross’ faeces, researchers found that a significant portion of their diet consisted of jellyfish. This is new because jellyfish had not previously been identified in either faeces or stomach. After all, they are transparent, and consist mainly of water.
From DNA to diet
The researchers who studied the diet of the albatross used a method that could identify species without depending on eyesight. This method, called “dietary metabarcoding”, involves reading the code of DNA (sequencing) extracted from the contents of the stomach, or, as in the albatross study – from the faeces.
Fact box: Sequencing means reading a DNA molecule. There are now many different sequencing machines with different ways of functioning, but they have in common that they describe the DNA code base by base, or letter by letter if you will. Unlike our alphabet, DNA has only four letters; A, T, G and C. |

By reading the letters of stomach-content DNA, one can be left with millions of sequences with small variations in the code, such as “AATGGCTACGGA” and “AATGACTACGGA”. Understanding which species the DNA molecules come from is nevertheless demanding, and here we need help from the world of computer science. By running advanced software on supercomputers, we can compare the millions of sequences we find in the sample against sequences of known species stored in databases. Since the sequences are more or less unique to each species (due to the small variations in the letters used), we can thus determine where they come from, and form a picture of which species compose the diet.
Examples from the Arctic
Some of the most important key species in the marine Arctic are found in a genus of copepods called Calanus. These are primarily considered grazing animals – that is, they feed on algae. Nevertheless, recent studies that have also utilized the new method, suggests that they also eat larger animals. DNA from bristle worms (Polychaetes) and arrow worms (Chaetognatha) has been found in the stomach of the Arctic copepod Calanus glacialis. In a similar study, it has been found that the more southern copepod species Calanus finmarchicus can eat jellyfish and nettles.
This is new and exciting simply because, bristle worms and arrow worms have been considered unlikely prey for a much smaller Calanus. It should be mentioned that Calanus probably feeds on the younger life stages of the larger animals, such as eggs or larvae. In that sense, the classic paradigm that nutrients move from small to large will remain valid. That is however not the point, and by using the DNA method, we have seen that nutrients and energy migrate not only upwards to higher trophic levels, but that they can also be transferred down the ladder. Thus, we see that the linear model (from small to large) does not always fit with the flow of nutrients that takes place.
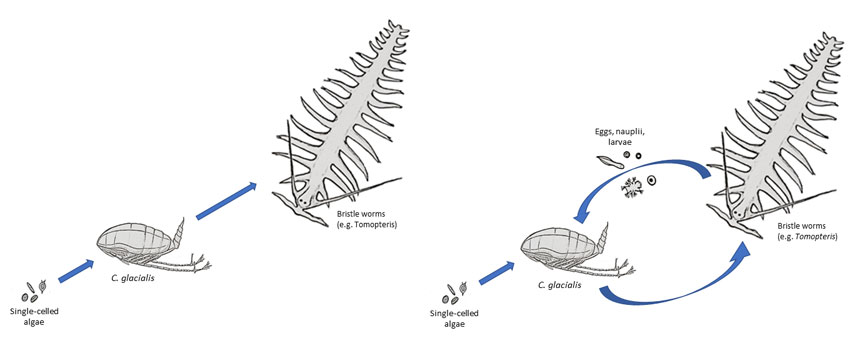
We still know very little
A common denominator with these studies is that they contribute to new knowledge, that in turn reminds us that we know quite little.
Therefore, in the large research project The Nansen Legacy, we are interested in utilizing this new technology when we go out into the Barents Sea. By studying small but numerous zooplankton such as Microsetella, we hope to be able to contribute to an ever better understanding of how nutrients and energy move in these waters. Could it be that they, like their larger cousins from the Calanus genus, feed on eggs and larvae of larger animals?
References:
- C. McInnes, R. Alderman, M. A. Lea, B. Raymond, B. E. Deagle, R. A. Phillips, A. Stanworth, D. R. Thompson, P. Catry, H. Weimerskirch, C. G. Suazo, M. Gras, S. N, Jarman: “High occurrence of jellyfish predation by black-browed and Campbell albatross identified by DNA metabarcoding”, Molecular Ecology, 16 August 2017.
- C. Cleary, J. E. Søreide, D. Freese, B. Niehoff, T. M. Gabrielsen: “Feeding by Calanus glacialis in a high arctic fjord: Potential seasonal importance of alternative prey”, ICES Journal of Marine Science, 25 July 2017.
- D. Yeh, J. M. Questel, K. R. Maas, A. Bucklin: “Metabarcoding analysis of regional variation in gut contents of the copepod Calanus finmarchicus in the North Atlantic Ocean”, Deep Sea Research II, 21 January 2020.